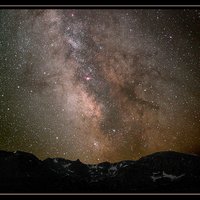
Sep 6, 2023
Transcript
[RADIOLAB INTRO]
LULU MILLER: This is Radiolab. I'm Lulu Miller. Today we're romping through some of the strangest places in outer space: black holes, multiverses and more. We've got two conversations that will stretch and spin your understanding of the starry soup in which we swim. First one comes to us from senior correspondent Molly Webster. She is in conversation with the astrophysicist Priyamvada Natarajan. Here we go.
PRIYAMVADA NATARAJAN: I am Priamvada Natarajan, and I'm an astrophysicist.
MOLLY WEBSTER: So maybe you could just start by telling me when we started thinking about black holes.
PRIYAMVADA NATARAJAN: The term 'black hole' actually comes from an infamous prison in Calcutta.
MOLLY: No way!
PRIYAMVADA NATARAJAN: Yeah! So on the 20th June, 1756, like, the ruler of Bengal then, his name was Siraj-ud-Daulah, he captured Calcutta back from the East India Company. And so he had all the troops, right? And he imprisoned them overnight in this tiny room, a prison where supposedly 146 of them went in.
MOLLY: Oh my gosh!
PRIYAMVADA NATARAJAN: Cramped in this hot, humid place with no water, and that only 23 survived. I mean, I don't know whether that number is actually accurate, but the thing is a large number of them actually passed away. So that was called—you know, it was the point of no return, so it was called the black hole of Calcutta. And this predates our scientific understanding of black holes by several hundred years.
MOLLY: Yeah, because I had heard that we started using the term 'black hole' scientifically, like, in the 1960s, but you're saying even before that it was around.
PRIYAMVADA NATARAJAN: Yes. So in 1887, there was a fire in Opéra-Comique building in Paris, and the New York Times referred to it as, you know, an 'immense black hole,' because everything was obliterated and nothing could be retrieved from that fire. Everything was destroyed. And a New York Times reporter, he mentions it as an immense black hole.
MOLLY: Hmm.
PRIYAMVADA NATARAJAN: You know, Edgar Allen Poe mentions the black hole of Calcutta when he writes one of his scary stories called The Premature Burial. So yeah, this comes again and again in popular culture, well before the scientific idea of a black hole and its properties were established.
MOLLY: It's interesting that, like, humans felt the concept of a black hole.
PRIYAMVADA NATARAJAN: Yeah. It's the imagination, right? It's the power of our imaginations, right? That our senses—I mean, you know, we view the world visually, and that fails when you don't have light. So we are sort of lost. And so I think there's fear that we associate with darkness, right?
MOLLY: It almost makes me wonder, when we first started thinking about black holes, in a scientific sense, if there was, like, a fear of them, like, if that's the phrase that we put on them.
PRIYAMVADA NATARAJAN: Right. I think fear and repulsion. And that's where the next Indian connection comes in, right? So the person who really proposed the astrophysical concept of a black hole as the end of, you know—the corpses that massive stars in the universe leave behind was Subrahmanyan Chandrasekhar. So he was this brilliant young man who came to England, to Cambridge to do his PhD. And on his voyage, he actually figured this out. You know, he married the then current understanding of stellar physics with the newly emerging quantum mechanics, and he was able to figure out that the end states of stars—stars, you know, live out their lives, they burn all the fuel, the hydrogen and the helium in their cores, and then they eventually, when they exhaust their fuel, they have—they end their life. And the way in which they end their life depends on the birth mass of the star, this mass that it was born with.
PRIYAMVADA NATARAJAN: And he figured out that stars that were born with masses maybe eight to ten times the mass of our sun will have a weird fate, like gravity would completely take over at the very end and they would collapse into this very, very dense object, which would be a black hole. And stars that were born with lower masses would end up in their lives as neutron stars. And those are just very dense. They're sort of the dense cousins, but they're not as enigmatic in their properties as black holes.
PRIYAMVADA NATARAJAN: And he presented this at a Royal Society meeting. And Sir Arthur Eddington, who was one of the most distinguished astrophysicists of his time in the world, Eddington speaks right after him and completely decimates him. And, you know, says, you know, his physics is wrong, his calculations are wrong. You know, Chandra is stunned at this public humiliation. Nobody else really stands up for him because of who Arthur Eddington is, you know? And it took a long time for the scientific community to accept this idea. The reason Eddington vehemently opposed it was he had a competing theory of stellar death. And of course, it is not lost on anyone that this was 1935. Remember, India was still a British colony and Chandra was a British subject.
MOLLY: So when did then Chandra's ideas start to become accepted?
PRIYAMVADA NATARAJAN: Eventually, you know, the two things, the two odd things that happened that really got the idea of black holes accepted as it were is, you know, during World War II when they were doing the bomb calculations. It's really strange, right?
MOLLY: I know, I was like, I did not expect you to go to World War II.
PRIYAMVADA NATARAJAN: I know!
MOLLY: But I am ready.
PRIYAMVADA NATARAJAN: Yeah. So the explosion of a star and the explosion of a bomb, it's the same equations.
MOLLY: Really?
PRIYAMVADA NATARAJAN: Yep. And they realized that a-ha! So now there was—you know, you could see how this gravity could just take over, and you could form this very, very compact kind of singular object. So there was this one line of evidence that showed, oh, this kind of thing could happen in the universe, right? The other thing, of course, as with all science, is sort of empirical discovery, right? Was the discovery by Jocelyn Bell of pulsars, these pulsating neutron stars. So, you know, so now that neutron stars were detected, you know, it kind of ratifies that there should be. Why not? Why you would ...
MOLLY: Then maybe our other guesses were also right?
PRIYAMVADA NATARAJAN: Exactly. And there were calculations, right? I mean, there were sort of serious computations. So I think that then added heft. And then later, one definitive way, empirical way to know and detect the presence of a black hole is the detection of x-rays from the gas that is being pulled around. For example, a supermassive black hole, like the one at the center of the Milky Way, the way you would see it and the way we have seen it, that image that you've seen, the doughnut image, and the glowing—the orange stuff that you see is the gas right around the black hole that is being pulled in by the force of gravity, and it's being sped up and heated up and it starts to glow. That's what we are seeing there, right?
PRIYAMVADA NATARAJAN: So if you look at the Milky Way, the mass of the black hole at the center of the Milky Way is four million times the mass of the sun. And the mass of our galaxy, of the stars in our galaxy, and dark matter in our galaxy is 1212 times the mass of the sun. So the black hole is really tiny, right? It's tiny tiny.
MOLLY: Wow!
PRIYAMVADA NATARAJAN: So it was then believed that because they are so tiny in terms of the overall mass budget of a galaxy, even if they're sitting at the center of every galaxy, which by the way, was discovered when I was in graduate school in 1998, nearly every galaxy in the nearby universe appears to harbor a black hole.
MOLLY: And what is—like, how many galaxies are in the nearby universe?
PRIYAMVADA NATARAJAN: Well, it depends on what you mean by 'nearby.'
MOLLY: [laughs]
PRIYAMVADA NATARAJAN: But, you know, one could easily say billions. You know, one could easily say billions.
MOLLY: So then billions of black holes.
PRIYAMVADA NATARAJAN: Yup.
MOLLY: Just at the center. Just if we're talking about centered black holes, billions.
PRIYAMVADA NATARAJAN: Right. And then yeah, they're littered everywhere. So this is super massive black holes in the centers, right? So recent theoretical work from my research group has shown that there's actually in the Milky Way, for example, there are probably another 12 supermassive black holes that are littered, that are wandering around, that are not at the center, that were kicked out of the center, right, over time.
MOLLY: I don't understand that. How—how do black holes float? A floating black hole to me is as if I was on a mountain and a rock started rolling down, and it was like—I'm like, "Oh my God, this rock is gonna hit me like an avalanche. This rock's gonna hit me and annihilate me." Does not a floating black hole in a galaxy—isn't it the avalanche of the galaxy?
PRIYAMVADA NATARAJAN: Well, not quite. So galaxies assemble in our universe. The way structure forms, you form the smallest galaxies, and they smash into each other, they merge into each other, and then they make bigger galaxies, okay? So this is how things hierarchically assemble. So if you imagine the two galaxies that are actually little galaxies that smashed into each other were already seeded with a black hole, right? So the stars merge upon collision. The dark matter kind of rearranges itself, because the dark matter, we believe, is made up of stuff that doesn't actually collide. It's collisionless, right? It's just held by gravity. And the black holes have to merge, or one of them will kick the other one out, depending on if these masses were actually not equal and they were really disparate, right? So you could kick out a black hole.
MOLLY: I think of black holes as rooted to a spot, and somehow they really change for me when they're floating.
PRIYAMVADA NATARAJAN: So, you know, black holes are a place that has a place in the universe, a place in which they warp the space time around them so extremely, right? And they have intense gravity. But, you know, it's useful conceptually to also think of them as objects, as, you know, very compact, dense things, right? And if you think of it that way, then you can kind of understand how—so, you know, black holes are actually spinning, too, right? So you can imagine they're kind of ...
MOLLY: Like the most chaotic cartoon characters I've ever heard. Yeah.
PRIYAMVADA NATARAJAN: They could spin and twist up space time around themselves, right? So I think it's useful to think of—you know, to kind of move between thinking of black holes as places and as objects.
MOLLY: As it's moving, is it reshaping the galaxy and forcing a different type of evolution with the stars that it comes near?
PRIYAMVADA NATARAJAN: So depending on whether this wandering black hole, like the details of how the collision happened, it could drag some stars with it, and it could have its own little bevy of little trail of stars that it's kind of held onto. And it doesn't move that fast. I mean, it's not gonna be moving at speeds of light or anything because galaxies themselves are kind of tumbling at velocities that are 200-ish kilometers per second or so. So these things are not going to be whizzing around anywhere near the speed of light or anything like that. We don't yet have definitive evidence for the detection of a fully wandering population.
MOLLY: Do we know if they'll ever settle somewhere? Like, I'm almost thinking of, like, a migrant peoples or a cult. [laughs]
PRIYAMVADA NATARAJAN: You can think of it as the attraction of gravity, something like a bowl, and this guy will be sort of a wandering black hole. Unless it's been kicked out with a huge speed, in which case it would exit the galaxy and would be completely free floating, right? So that's possible, too. But black holes, eventually, they will settle to the center. They'll be pulled in by the gravity, because the biggest one will be in the center. But the black hole, even at the center of our own galaxy is not sitting still, right? It kind of wobbles a little bit here and there, like, a very small wobble, but it does.
MOLLY: My gosh, I wonder if we ever feel the wobble.
PRIYAMVADA NATARAJAN: But, you know, we don't feel the earth rotating, do we? So forget it, Molly. We're not gonna feel the wobble. [laughs]
MOLLY: I don't know, I'm just thinking Chandra was out in that boat. He did a bunch of math. He came up with black holes. Maybe he felt the wobble.
PRIYAMVADA NATARAJAN: But, you know, when you think that you have equations in mathematics that can really describe the natural world, I mean, surprisingly well, right? I mean, that is still a kind of a miracle. Like, why math as a language works so well in describing the phenomena we see in nature, right? And I think part of—tied in with all of that is like the so-called information paradox, which is no longer a paradox. The fact that what really happens when light, matter, anything crosses this sort of sacred boundary of a black hole called the event horizon, a point of no return, right? And I think that's where I can now bring up that Hawking story that you ...
MOLLY: Oh, yeah. I'll just jump in here to say that before the interview, I told Priya I wanted to talk to her about Stephen Hawking and this idea that he came up with that's now called Hawking radiation, but it has to do with what happens to stuff after it falls into a black hole.
PRIYAMVADA NATARAJAN: So, you know, Hawking, I knew him in Cambridge, and I've had the real privilege of talking to him about my thesis work, some of which was on black holes and some of which was on dark matter. And I think one of the analogies that he had to think about information and whether you can retrieve it or not, was that imagine you had an Encyclopedia Britannica. So you have the full volume in front of your hands. You have it in your hand, you're looking up New Haven, Connecticut, all the details of population, geography, et cetera.
PRIYAMVADA NATARAJAN: Now you put the encyclopedia in a glass—in a box, okay? In a box. And you burn it. And the box is really tight. Nothing leaves the box, right? So all the remains of the encyclopedia, all the ash, the carbonated ash, is sitting inside that box. Nothing's left that box, right?
MOLLY: Mm-hmm.
PRIYAMVADA NATARAJAN: So at some level, there is no information that has been lost as such, right? It's still there. It's just that it's no longer written in a language in English that we understand. And we also don't know how to retrieve that information from char, right? From char, charcoal. We just don't know how to extricate that information. So that's the situation, that the information is most likely not lost, it's just that we do not yet know how it is stored. Like, how it mutates, like, once it crossed the event horizon, how does it transmute? What does it transmute into? In this analogy, it transmutes into, like, ash, right? But we A) don't know how and what it transmutes to, and we don't know how to recover the information from whatever it has transmuted to.
MOLLY: And then how does sort of his idea of Hawking radiation, like, tie into the metaphorical ash?
PRIYAMVADA NATARAJAN: Oh yeah, so the metaphor—so Hawking radiation, basically, black holes should be radiating energy off, because, you know, vacuum is not completely empty. So you have particles and antiparticles that are whizzing around. And occasionally around a black hole, you could have an antiparticle that goes, crosses the event horizon, but its particle equivalent is on the other side, right? And so, you know, you have this kind of process by which you have energy coming—supposedly coming out of the black hole, right? So there's energy loss, there's a temperature, and there's therefore evaporation. You can associate a temperature with a black hole. You can associate an area with the black hole, and you can associate an entropy with the black hole. And so therefore, an object that has entropy and has a temperature will evaporate, okay? But it takes a long time for evaporation, so none of the black holes that we are seeing and we are measuring in the universe, stellar mass black holes or supermassive, they are not gonna evaporate anytime soon. So those are pretty stable. But any little guys that formed, you know, primordial black holes that formed right after the Big Bang, if they were less than 1015 grams, they would have evaporated away by today.
MOLLY: Hmm. This idea that there's a particle that could escape out of a black hole, that there was something inside the black hole that knows about the inside of the black hole, and then it gets out to the universe, I always thought that image was a really beautiful, almost like, human metaphor, because I thought, oh, you know, it's like we're all little black holes just bumbling around and, like, we're giving off some hints of, like, what's happening inside of us and who we are and, like, what we've come across. And sometimes that's verbally, and sometimes it's non-verbally. And now you're saying, to really add to the poetry, if you give off enough, you disappear. [laughs]
PRIYAMVADA NATARAJAN: [laughs] No, but also we do—we do disappear, you know, in the wink of an eye, right, in terms of the age. I mean, our lifespans are, like, tiny compared to any other cosmic time scale, right? So we're like, it's a blink of an eye. We are here and we're gone. It really says something about—you know, it captures the complexity of human beings, right? And the fact that you never really know everything that's there. You can never really know somebody completely, right?
MOLLY: That was Priyamvada Natarajan, an astrophysicist at Yale. Thanks, Priya.
LULU: If you'd like to dig into her work, check out her book Mapping the Heavens: The Radical Scientific Ideas that Reveal the Cosmos. And stick around, because next up, we're surfing through the multiverses. You knew it was time. We gotta do it. Stay tuned.
LULU: Radiolab. Lulu. We are journeying through space this hour. And up next we have a conversation with Robert Krulwich, longtime host of Radiolab, and theoretical physicist Brian Greene. They spoke together as part of the 92nd Street Y's long standing lecture series. Here we go.
ROBERT KRULWICH: The man you are about to meet is, he's a professor at Columbia University of mathematics. He's also a professor of physics at Columbia University. He's also the director of the Institute for Strings, Cosmology and Astroparticle Physics at Columbia University. He's also a best-selling author, he's also done work in what's called 'mirror symmetry,' which means I'm not sure what, exactly.
[laughter]
ROBERT: It involves relating two different Calabi–Yau manifolds, relating the conifold to one of the orbifold, which I'm sure you know all about. He's a Rhodes scholar. He's the author of The Elegant Universe, so basically he's not a dumb person, not at all. But he does, however, believe the most peculiar thing: so if you ask him—and I will, he believes that if you travel from this room, from this auditorium on 92nd Street and Lexington Avenue, in pretty much any direction at all, but if you have to go really, really, really far—light years upon light years upon light years out, you will, if you travel far enough, eventually find a galaxy that looks—and I mean exactly like our Milky Way. And in that galaxy, you will find a planet that is an absolute copy, a dead ringer for our planet Earth. And on that planet, you will find a city that is uncannily down to the hairs on the city rats, exactly like this city, New York City. And in that city, there will be another 92nd Street YWYMHA, with a building that has another auditorium in it that is exactly like this auditorium here.
ROBERT: And if you think that's an unlikely coincident, I'm just getting started, because I think Brian Greene will also tell you there is another professor who also teaches both mathematics and physics at a school that just happens to also be called Columbia University, who's also the director for Institute for Strings, Cosmology and Astroparticle Physics, who also just happens to be named Brian Greene with an E on the end like this one one out here. And in these two rooms, these two identical professors with their identical paths are going to be talking to yes, an identical audience watching, which is an exact copy of every one of you sitting here in this room right now, with all your histories and all your memories. Brian Greene believes there's not only one doppelganger room with a doppelganger Brian Greene and a doppelganger audience, he believes there are countless doubles of us in every direction out there in the great beyond. And what's more, there are rooms exactly like this one with just you missing, or just me missing, or the color of my shirt slightly different. In other words, in a big enough cosmos, in an infinite cosmos, Brian Greene thinks that anything you could imagine, almost anything that can be out there will be out there. Really out there. To which I say, oh, come on! Does he really believe this? And if so, why? So let's ask him. Let's welcome the local homegrown version of a man I like to call Brian Greene.
[applause]
ROBERT: So far so good? Did I get it right?
BRIAN GREENE: More or less. There's an assumption you left out, but we can get to that.
ROBERT: Okay. Well, let's talk about two words: infinite and universe. Let's do infinite first. We're gonna start infinite by discussing what you have—in a manuscript you're working on now, you've described it as the Imelda Marcos wardrobe problem. So we have a woman here who does not like being seen in the same outfit twice. So tell us how she solved it.
BRIAN GREENE: The issue that you're referring to is the following: if you have a finite number of distinct ways, in this particular case of dressing, and you have, say, an infinite number of days and evenings on which you have to step out, then it's pretty clear that you're going to have to repeat an outfit once, twice, in fact innumerable times, because you only, say, had 10 different outfits. Or in the case of Imelda, the example there, I forget how many ...
ROBERT: 500 dresses and 1,000 pairs of designer shoes.
BRIAN GREENE: Right. There you go. So you can envision the number of distinct combinations of dresses and shoes. Very easy to figure it out. Just 500 times 1,000. And then if there are more than that many days, then that individual, that Imelda is going to have to repeat, is going to have to repeat a given outfit if there's, say, infinitely many days and only finite number of outfits. Simple idea.
ROBERT: All right. [laughs] So now let's just use that logic and apply it to just a different kind of system. Imagine a cosmos which has infinite space. Now the key thing here, I think, is that the stuff in the cosmos, you believe, has some kind of finite set of rules. So let's take her, this woman, in the—what are you? Are you in the pinkish kind of—red. Okay. So she is a compilation of atoms which follows certain rules. Her memories are, I assume you think also atoms?
BRIAN GREENE: I do. Not everybody agrees that every aspect of your personality and your conscious being is reducible to a configuration of the atoms that make up your brain, but I certainly believe that. And that's ...
ROBERT: Dreams, memories.
BRIAN GREENE: That's right. There's nothing else. All you are is a bag of particles acting out the laws of physics. That, to me, is pretty clear, however distasteful.
ROBERT: Wow! Say you.
[laughter]
ROBERT: The kiss of God we just don't include in this arrangement.
BRIAN GREENE: You can if you'd like. I just don't see any particular need for it. So for this discussion ...
ROBERT: [laughs] So if she is just a large number of atoms in a particular array, but let's say she can—we can travel infinitely and look at all of—and we're talking here, what, I guess, about particles or about forces or about ...?
BRIAN GREENE: You can reduce everything basically to particles. That's a fine way of phrasing the whole conversation. And the idea that you're getting at is indeed the fact that if the universe is infinitely big, then the number of distinct configurations of particles actually is finite. And if the universe is infinitely big, then just as your coin tosses have to repeat, the configurations of the particles will have to repeat. So you go sufficiently far out there, and the configuration of particles will repeat, and will indeed make a configuration of particles that looks just like this room.
ROBERT: Looks on the outside, or is? So she had a dream last night about a black cat walking on a fire escape. She is also deeply in love with the man next to her, and they share an enormous number of experiences together. So we have a lot of atoms there doing very different things—if indeed they're doing these things at all. You say that that combination, the intimate inside and outside combination here, will be found elsewhere again and again and again.
BRIAN GREENE: Yes, I'm actually saying both. I'm saying that the outside will be found with a distinct inside many places out there, but the outside with the same inside will also be found out there, because again, it's just another configuration of the particles. And since there are only a finite number of distinct possibilities, it's a huge finite number. It's a gargantuan finite number, but it's a finite number. And if you go sufficiently far out, there's just so many distinct configurations that there can be if they're finitely many, so if you go sufficiently far out, you have to repeat.
ROBERT: But won't you run into a quadrillion people almost like her?
BRIAN GREENE: Oh, absolutely. It's much easier to reproduce this—this woman in the first row with one particle in a different place or two particles in a different. Those are much easier because there's less constraint. If you want to reproduce this individual completely, every single particle has to be in the right place. That just means you have to go a little further.
ROBERT: Now you—I know we're speaking here, and this makes perfectly logical sense. It just makes absolutely no practical sense, obviously. But I'm also wondering whether it makes sense to you as a person. Do you literally think that there are two people exactly like us with our dreams, our thoughts and da da da, in some other stage right now, or whatever you'd call 'now,' over and over again right now, all over the universe? Or do you just say that?
[laughter]
BRIAN GREENE: Well, there's a key element that we have been assuming, and we've been assuming that the universe is infinitely big. And we don't know that the universe is infinitely big. It could be that you go on for some distance, and you walk and you walk and you wind up coming back from that direction, sort of like the surface of the Earth. It has a certain size. It's not infinitely big. It doesn't have an edge. You can't fall off, but nevertheless, when you keep on going, you return to your starting point. It could be that the universe has that kind of a shape. The data seems not to support that at all at the moment. The best astronomical data does not support that. It supports a universe that is flat as opposed to curved.
BRIAN GREENE: And if that's the case, it's still possible that the universe could be sort of like the surface of the Earth, in the sense that it might be like the surface of a video game screen. If anybody who plays Pac-Man or any of the other videos, you know that, you know, when Ms. Pac-Man falls off the right side of the screen, you know, she doesn't disappear. She comes back on the left side of the screen. That's another possible shape for the universe. It has a name, it's called a torus. And our universe might have that particular shape. Again, it's a finite shape, but you can't fall off. We don't believe you can fall out of the universe, so that would be a way to have a finite universe where you don't fall off. However, the other possibility is simply that it goes on forever. And we don't know which is right. The simplest one mathematically is that it simply goes on forever. And if that's the shape of the universe, then yes, I fully believe that we are out there in some notion of now having this conversation, not once, not twice, infinitely many times.
ROBERT: Well, aside from the deep creepiness—well, let's talk about the creepiness of that.
[laughter]
ROBERT: I mean, there is a notion that we all have that we are unique individuals, and that there is a real version of us. In my case, you're looking at him. So ...
BRIAN GREENE: You just said that a million, billion times over in the other universes.
[laughter]
ROBERT: Well, so where does that leave you? Do you just feel like you're copy number 418, or ...?
BRIAN GREENE: Personal identity in this context is very, very strange. There's literally no difference between me and the other guy out there. And that's okay with me. I don't really have a problem with that, but I do agree that it challenges your sense of what your personal identity is all about. You are the collection of your memories, which are ultimately just a collection of impressions in your brain, just a collection of particle configurations. And if that other individual has the same brain configuration because the particles are in identical state as they are in my head, that person is as much me as I am me.
ROBERT: So doesn't that automatically make you want to quickly run off the stage, hide under a pillow, do something that the other guy could never find out and then be different?
[laughter]
BRIAN GREENE: Well, I know that he'll have exactly the same thought, so it won't help me very much. But ...
ROBERT: That's really creepy, to be chased around by the—by the infinite number of doppelgangers so that everything you will to do, they also will to do simultaneously.
BRIAN GREENE: And there are infinitely others that will to do something slightly different. So there's just a richness to the world, but its complexion is somewhat different than what you had in mind. I think most of us have in mind that if the universe is infinitely big, you keep on going, you see new landscapes. Keep on going and see new stuff, new stuff, new stuff. That's our picture of what reality is in that kind of a universe, our universe. And I'm saying that the laws of physics don't support that notion. The laws of physics support yes, it's a rich world out there, but the distinct landscapes, the distinct environments, the distinct configurations that you can see are limited.
ROBERT: What about how far away is the nearest one to me?
BRIAN GREENE: Well, that's a good question. You can actually calculate that, and as you might imagine, it's a pretty big number, because ...
ROBERT: I'll give you a number from a guy named Max Tegmark at MIT. I have no idea what this means. What is 1010 to the 29th meters?
BRIAN GREENE: That's a big number.
[laughter]
BRIAN GREENE: And you know what it means to ...
ROBERT: It's not like, "Hi!" It's not a ...
BRIAN GREENE: Well, just to quickly say what that means. I think you're familiar, you know, with what 102 means. It's 100, just a one with two zeros following it. In this particular case, you take 1029—that's a big number. One with 29 zeros after it. And then you take ten to that number, which is that many zeros following 1029 zeros following the one. And it's so big that we don't have a name for—I mean, call it the Krulwich, whatever. I mean, it's a huge number, and it just is commensurate with the fact that even though we're saying that there are a finite number of ways that my particles—my electrons, my protons, my quarks, there's a finite number of distinct ways that they can be configured, it's a huge number, but in an infinite universe—let me just stress, in an infinite universe, 1010 to the 29th is really small. I mean, infinity is a hard concept to wrap your mind around.
ROBERT: Yeah, it is.
BRIAN GREENE: I mean, you take half of infinity, you've got infinity. You take a third of infinity, you take a tenth of infinity, you've got infinity still. So this number, however big it may seem by human standards, is just a little tiny blip in this infinite cosmos.
ROBERT: Well, there was one other assumption that we made, and maybe I should call attention to it. You said that all of the particles that make her up and all of the forces that join those particles are a finite group. Now how do you know that? All you know is what you can see here where we are. Why wouldn't—you know, who says?
BRIAN GREENE: Well again, there are underlying assumptions that the laws of physics that we know about here are the laws of physics that govern the universe everywhere. That's again, a rather simple assumption that we don't know to be true. Certainly, our observations of the universe suggest that the parts that we can see visually are governed by the same laws of physics that govern the phenomenon that we can see the world around us. That doesn't prove that if you go sufficiently far out, the laws might change. Simplest assumption is that they don't change—and that's certainly what I am assuming. In some of those universes, there will be one lonely human, the only person that's formed out of the particles in that universe, and in a very tiny fraction, there'll be universes that look like ours.
ROBERT: Well, since you've slipped in this plural for universe, now 'universes,' because I don't know that I should just let you do that. Let's just do that. When you use the word. When I use the word 'universe,' I use it in the common sense that it includes everything that we know of or that we can imagine. That seems to not be even remotely what you're doing.
BRIAN GREENE: Well, we found that that notion of universe, however all encompassing it sounds, is a little too coarse to accurately describe modern thinking about how the physical universe actually works. And we find it more useful to have words that delineate the universe with finer characteristics than lumping it all together into everything that you can imagine, everything that's out there.
BRIAN GREENE: So for instance, we imagine the observable universe. That's the part of the universe that you can, in principle, see with a sufficiently powerful telescope, with a sufficiently powerful receiver, you'd be able to exchange signals with those parts of the universe. We believe that there are parts of the universe that are just beyond our ability to see because there's not enough time since the beginning for light or any other influence to travel from that distant location to us. So that's a part of the universe that's kind of separate from us. It's not within the observable universe. So some people like to think of our observable universe as one contained universe, and those other regions as in some sense other universes. They're not that distinct. In principle, you can get to them if you travel sufficiently far. They're just regions that are beyond our ability to exchange influence with today.
ROBERT: How much space can we see? What's the observable?
BRIAN GREENE: Roughly 42 billion light years in any given direction.
ROBERT: So is there a place out there where conceivably what is predictable over here changes out there? Is there—is there any neighborhoodness ...
BRIAN GREENE: Where the laws actually might be different.
ROBERT: Yeah, let's say that.
BRIAN GREENE: Yes, absolutely. So in fact, when we study modern cosmology with greater intensity, we find that it's probably likely that if you examine the universe from, say, a God's eye view, so using that capital-U universe that you started with, if you can sort of see in some sense the whole thing, not just what we individual humans have access to, if you can see the whole thing, then you'd probably find, according to a theory called inflationary cosmology that we can discuss if you'd like, but according to this theory, the likely complexion of the universe is that there are a variety of bubbles, bubble universes. Ours is just one of this vast cosmic bubble bath of universes. And in the different bubbles, the laws of physics can appear to be somewhat different from the laws that we are familiar with.
ROBERT: When you say they can be different, so are you talking about all hydrogen but no oxygen, or are you talking about, "Oh, actually, we don't have gravity here. We don't do gravity. We don't actually have a weak force."
BRIAN GREENE: It's more the former. But actually, the distinction between those two examples is not as clear cut as you would envision. So for instance, if you talk about not having the nuclear forces, which is what you were alluding to, it's possible that the nuclear forces can be in our universe as we measure them and in that other bubble universe, but in the other bubble universe, they might be so weak compared to their strength in our universe that they don't have much of an impact. The impact might be infinitesimally small compared to the impact in our world. So for all intents and purposes, there might not be a nuclear force in that universe compared to the nuclear force in ours.
ROBERT: Well, now this is getting a little complicated. So we're here with a set of rules and a set of operating systems that we have somehow magnificently begun to figure out. And then you say there might be a neighbor where all of the principles that have organized life and existence here may not be operating quite the same way. But I guess I kind of thought that we here eventually attached to them there. So these bubbles you talk about ...
BRIAN GREENE: These are different kind of bubbles, bubbles where we don't attach. It's as if the universe is a big block of Swiss cheese. And I'll just stop right there. Thank you.
ROBERT: [laughs]
BRIAN GREENE: No, it's like a big block of Swiss cheese where the holes are the universes that we're talking about. So everything that we know about is one hole in the cosmic Swiss cheese, but there are many other holes in the cosmic Swiss cheese. And the point is when we study this picture that emerges from studying the cosmological data, not from an overworked imagination, we find that the meaty part of the cheese—sort of a bad vegan metaphor there, I guess. But, you know, the white—the white part of the cheese expands so quickly that the bubbles, the holes in the cheese are pushed apart at an enormously fast rate, faster than the speed of light, and that's what prevents one from even, in principle, going from one hole, one bubble, our universe, to another hole, another bubble. So our universe and the other universes are all embedded in this environment, this cosmic Swiss cheese, but we can't traverse the cheesy part because it's growing so enormously quickly.
ROBERT: But—oh, dear!
[laughter]
ROBERT: See, now let's go with your metaphor here. So we have this big Swiss cheese of a mega something of the place.
BRIAN GREENE: Multiverse is actually the word that ...
ROBERT: Multiverse. All right. So now I—we're inside, we're in a space. So this is your cheese hole now?
BRIAN GREENE: Yes.
ROBERT: Okay. So I'm living in there, you know, and my universe is getting bigger and bigger. So this Swiss cheese has a hole that is growing.
BRIAN GREENE: The hole is growing larger.
ROBERT: The hole is growing. And you're saying that the cheese ...
BRIAN GREENE: The ripe part of the cheese is also growing, and it's growing faster than the rate of which that hole is growing.
ROBERT: Wow!
BRIAN GREENE: So there's bigger holes, but more cheese.
[laughter]
ROBERT: So if you're in your—this particular evening is brought to you by the American Dairy Association. And we'll be doing it in Wisconsin next week.
BRIAN GREENE: [laughs]
ROBERT: So the reason I can't talk to you if you're in your hole and I'm in mine is I'm here growing, you're over there growing, but the space between us, we would be flying to either side of this stage.
BRIAN GREENE: Yes.
ROBERT: Faster than the speed of light, you say?
BRIAN GREENE: Yes. Faster than the speed of light. Sometimes people say, "Well, how is that possible? Nothing goes faster than the speed of light." And the thing to remember is that when Einstein established that nothing goes faster than the speed of light, what he really established is that nothing can traverse space at a speed greater than the speed of light, but his equations full well allow space itself to grow at any speed. Bigger than the speed of light is perfectly fine.
ROBERT: Okay, I'm gonna have to keep summing up, because otherwise I'm gonna get completely lost. So the universe then is a series of expanding empty spaces inside a web of some kind—we'll call it the big cheese. And the webs are growing. What I don't still understand is where did these holes come from? In Swiss cheese, I have no idea where those holes come from either.
BRIAN GREENE: [laughs]
[laughter]
ROBERT: I consider this the dual hole problem myself. Well, could you address, please, the spatial holes, and we'll leave the dairy holes for another occasion?
BRIAN GREENE: Yeah, so in this version of cosmology, again it's called inflationary cosmology, what happens is in the beginning, whatever that means, space is filled with something that we call a field. An inflaton field is the name of it. And a field is very much like an electromagnetic field. I think we're all pretty much familiar with that idea that there are electromagnetic waves going through this room. That's how you can use your cell phone to talk to somebody far away: the electromagnetic waves are suffusing space. Those electromagnetic waves are fields, electromagnetic fields. This inflationary theory invokes a new field, not the electromagnetic field, that fills all of space. And it has the amazing property that by virtue of filling space, it causes space to be filled with repulsive gravity. That's what this field does. Repulsive gravity pushes everything apart.
ROBERT: So this is—gravity, normal gravity brings things together. This is the ugly twin.
BRIAN GREENE: That's right.
ROBERT: Get out of my way, gravity!
BRIAN GREENE: That's right. And Einstein himself is the one who found this. This is an old idea, repulsive gravity. It goes back to 1917, even though it's not widely appreciated that gravity can be repulsive. And in this environment of this field filling space, gravity is repulsive, drives space apart. As space is driven apart, it turns out that there are regions where this field drops to zero value. It quantum fluctuates to zero value, and those are the holes. So we, our universe, is simply a fluctuation in this field where it had a high value and it dropped to a low value—thankfully—then giving rise to the universe that we are familiar with. So the cheesy parts of the Swiss cheese are the places where the field has a finite value, and the holes in the Swiss cheese are where the field has dropped to zero value.
ROBERT: Okay. So this leaves me then—infinity I get. On the universe side, what we end up with then is very distinctly different neighborhoods. Each of these bubbles can have its own character. So some of them, you could have atoms that come together happily and form chains and then form us, and then even give rise to intelligent things. And other things you just get, like, sand or—I don't know, be really boring for us.
BRIAN GREENE: Yeah. Yeah, exactly.
ROBERT: So then the universe has got—is this an infinite universe, this multiverse?
BRIAN GREENE: Well, that's a good question because ...
LULU: Stick around, because Krulwich and Brian Greene are going deeper into the multiverse after this quick break.
LULU: Radiolab. Lulu. We are jumping right back in where we left off with theoretical particle physicist Brian Greene and curious retired radio host Robert Krulwich.
ROBERT: Is this an infinite universe, this multiverse?
BRIAN GREENE: Well, that's a good question. So there are a number of kinds of infinities that now come into the story. Number one, you can ask how many different bubbles are there? How many different holes in the cosmic Swiss cheese are there? And indeed, this is a process that we believe to be eternal. And therefore, there would actually be an infinite number of these little bubbles with our bubble being just one. And now you might say, "But wait a second, you started off this conversation talking about space that we have access to going on infinitely far. If our universe is a bubble, no matter how big, it doesn't go on infinitely far. It has some size to it." To someone on the outside, it looks like this bubble has finite size. To anybody on the inside, it looks like it has infinite size. So the conversation that we had 15 minutes ago about a universe that goes on forever applies here, even to each of those individual bubbles. So in each bubble, space goes on infinity, so there are an infinite number of copies of us having this conversation.
ROBERT: Inside our ...
BRIAN GREENE: Inside a single one of these bubbles. And then you got infinite number of bubbles where anything else can be going on. There's a danger that people say, "Oh my God, this is so far out and nuts. This is ..."
ROBERT: It's pretty far out and pretty nuts.
BRIAN GREENE: This is a very minimal, a very minimal approach to describing observations that we have, observations of the early universe by looking deeply into space can only be understood, largely speaking, in the context of the frameworks that we're discussing here.
ROBERT: You're saying to me that all these nutty ideas come from very, very serious premises.
BRIAN GREENE: Yes. They don't come from people saying, "Let's think up a nutty idea and examine its consequences." They come from people saying, "Let's do measurements of the universe, and try to come up with theories that explain those measurements."
ROBERT: I'm not even gonna ask you what measurements those were.
BRIAN GREENE: No, literally that's not hard to describe. It's literally measurements of the temperature of radiation that we believe is left over from the early stages of our bubble universe. You measure the temperature over there, the temperature over there and so forth, and you get certain numbers and you try to explain them with theoretical equations. And the framework that is able to best describe them winds up entailing all of the additional structure that we're talking about. It comes along with the theory. It's not something that you put in from the outside.
ROBERT: Well, I'm gonna get as weird as I know how to get here based on what you've told me before. In addition, imagining now a universe in which there's a bubble in which we have carbon and hydrogen and oxygen and all the forces that we're familiar with, and we have planets and mass and clouds of gas, and you and me, over and over and over again, that universe. And then we have another universe not too far over in that direction in which very little happens, another one in which we get halfway there, another one in which very odd things happen. What about this: one of these bubble universes produces super intelligent computer geeks, intelligent beings, and what they do is they do The Matrix. They come up with a supercomputer, a fabulous supercomputer that creates a universe so spectacularly realistic that the critters in that phony fake universe think their law. If you can give me our universe and weird universes and weirder universes, can you eliminate the possibility of totally fake universes?
BRIAN GREENE: Not only can't you eliminate it, it's actually quite likely.
[laughter]
BRIAN GREENE: I mean, you see in our universe, just using that as a benchmark, that we're getting closer and closer to being able to do exactly what you're talking about. Imagine technology 1,000 or 10,000 years or 100,000 years down the road, one presumes that if progress continues apace, that we will be able to create the kind of simulations, computer simulations, that to those bits that are being manipulated in that simulation appears to have a reality in much the same way that you're familiar from The Matrix. Now the place where the ideas that we're talking about come in is the following. If you ask yourself the following question: is it more likely that if I'm a being that sees a reality, is it more likely that's a real reality that came from the universe creating it as, say, one of these bubbles? Or is it more likely that I'm in a simulation?
BRIAN GREENE: And all we're talking about here leads to a rather interesting conclusion, because for the universe to create a reality that we're familiar with is a relatively unlikely course of events. Because the universe is creating all these bubbles, the particles are coming together in one way or another, randomly joined together according to the laws of physics, and only in some real tiny fraction will the configurations give rise to human beings, say, that see a world akin to the one that we see. That's rare. In one of those bubbles though, if you do have these super-intelligent beings, maybe they're not even super intelligent, just super expert at dealing with the technology of their time, if they can create simulated universes, it's a much more direct route to a reality that we would consider akin to what we experience. It's not random, it's individuals actually creating the simulation. In fact, they can create simulation after simulation after simulation after simulation.
ROBERT: Oh, no!
[laughter]
ROBERT: So the chances of us being in a naturally-occurring 'real' world might be smaller than the chances that we belong to the fifth grade project of a geeky kid in the planet Zantar.
[laughter]
ROBERT: I don't know how you live with this stuff.
[laughter]
ROBERT: And we've only just sat down, and you've already told me that we are doppelgangered up the gazoo. We therefore seem to have no real identity. Free will is an open question, and we're probably a fake.
[laughter]
ROBERT: Assuming that you believe these things to be true, doesn't it get you down?
BRIAN GREENE: No, I think it's incredibly exciting. I mean, to me, the most wondrous thing about science—and physics in particular—is the fact that through the power of thought and calculation and observation, you can be led to conclusions vastly at odds with what you would think based upon experience. I don't think there's anything more wondrous than that moment when you think the world is one way, and your equations, your math, your ideas, your theories begin to convince you that it is another way.
BRIAN GREENE: And we've seen this played out in the history of science over and over again. It's not something that gets you down, it's something that pumps you up. And if we are now perhaps learning that the very nature of reality at least needs to be questioned, it doesn't in any way establish that we are in some simulation, because it's more likely, perhaps, that we are in one—we could still be in a real universe—but to me, it's wonderful to get your sense of reality kicked, to get a kick in the head, and to at least be able to contemplate the real possibility that the laws of physics are suggesting that reality is not what you think it is. However diminishing that may make some people feel, I think we need to perhaps face up to the real possibility that the notion of having free will is an illusion. It's a useful illusion. It makes life interesting, and it's one that you need to put to the side, because you need to live as though you do have free will. But all there is is physics.
[laughter]
ROBERT: [laughs] Don't you—there are a lot of people, Paul Steinhardt, David Gross, Martin Gardner, who have some real problems with this multiverse concept, and I just want to run a few of them by you. One of them is: to conjure up a universe in which everything that could happen is happening, doesn't that break one of the basic rules of science, the keep-it-simple rule? Occam's razor? I mean, if you can—or I'll just quote Martin Gardner. "Surely the conjecture that there's just one universe and its creator is infinitely simpler and easier to believe than there are countless billions upon billions of worlds."
BRIAN GREENE: It does sound simpler in some ways. And I have to say, I'm gonna give you two answers to this issue. If it turns out that our future understanding eliminates this idea of parallel universes, multiverse theory, and winds up showing, ah, the way to think about it is this, there's one universe, we can calculate everything, it's uniquely determined by the laws of physics, I think I'd be happier with that outcome. So don't get the misunderstanding that I have some emotional connection to this idea. It's where we're naturally led by a variety of interesting ideas, and therefore needs to be taken seriously. But in terms of it being simpler to have one universe versus many, it really depends on how you define 'simpler.'
ROBERT: All right, so we're left with a bunch of choices. We are infinitely duplicatable. We live in cheese, in expanding holes. We might be a reflection of a computer. We might be a pale reflection of mathematics. We might be actually made of real stuff in the here and now. Or not.
LULU: That was Professor Brian Greene in conversation with Robert Krulwich. Check out Radiolab.org for more. And thanks as always. No matter what the physicists say, I don't believe you are infinitely duplicatable, listener. You are one of a kind, and our gratitude is vast for your listening.
-30-
Copyright © 2024 New York Public Radio. All rights reserved. Visit our website terms of use at www.wnyc.org for further information.
New York Public Radio transcripts are created on a rush deadline, often by contractors. This text may not be in its final form and may be updated or revised in the future. Accuracy and availability may vary. The authoritative record of programming is the audio record.